Smart structures seminar
At Cnam, Paris, July 3rd 2017, 3 p.m.
Andrea Bergamini, Edgar Flores Parra, Johan Frederik Toftekær
Exploitation of mechanical and electromechanical coupling mechanisms for wave propagation control
Andrea Bergamini
Mechanical Integrity of Energy Systems Laboratory, Empa
Swiss Federal Laboratories for Materials Science and Technology Dübendorf, Switzerland
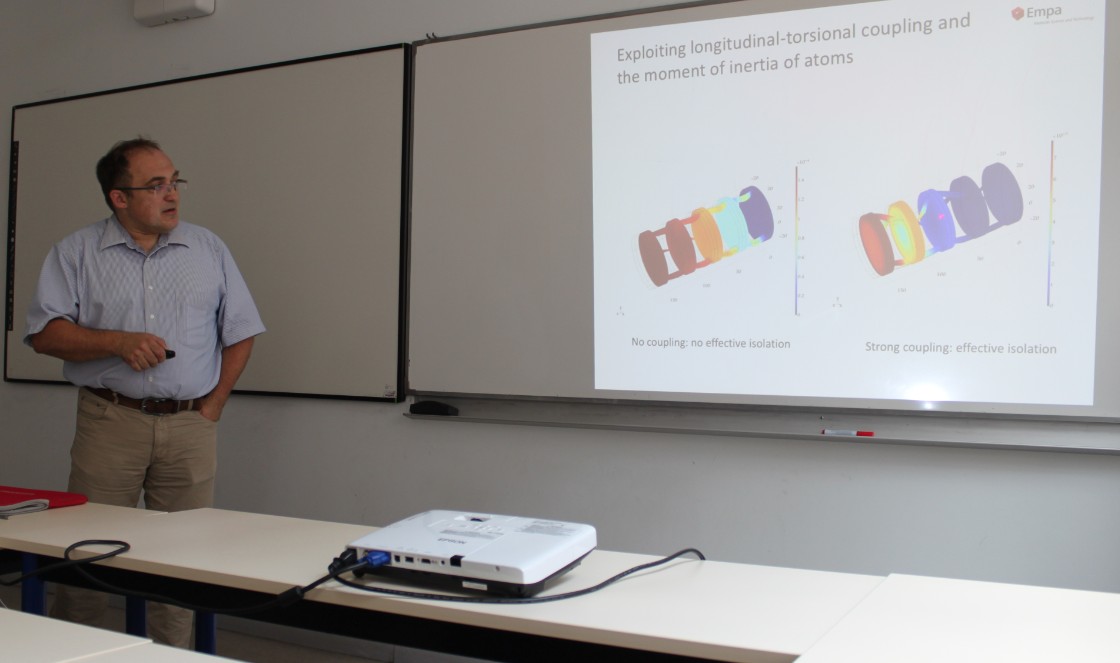
The formation of bandgaps in phononic crystals (PC) is object of extensive investigation by a lively scientific community. While achieving low frequency values for the position of the first bandgap is, in general terms, not an insurmountable challenge, the combination of material properties such as high stiffness, low density and reduced size of the unit cell, with low (in absolute terms) frequency bandgaps may well require some careful consideration.
For this purpose, our work has been focusing on exploiting different types of coupling between the power of mechanical vibration and other energy fields. In previous work, we have found that the relation between the mentioned material properties and the position of the first bandgap for a given mechanical PC geometry can be well described by the relation:
w* = fBG·L·( reff / Ceff )0.5, where fBG is the start frequency of the first bandgap, L, Ceff, reff are a characteristic length, the stiffness and the density of the unit cell, respectively.
w* appears to be a property related to the geometry of the unit cell. In an effort to design PCs with w* values below the ones achieved with resonant metamaterials, we have devised a novel implementation of inertia amplification based on coupling of the energy of longitudinal waves into rotational oscillation of inertia elements within the unit cell.
Further work has focused on exploiting the electromechanical coupling properties offered by piezoelectric materials to pursue the same goal of low frequency bandgaps in lightweight, stiff materials. Here we have considered exploiting the ability of resonant piezoelectric systems to realize "zero-stiffness" elements to tailor the stiffness matrix of structures as a function of frequency. Another approach is based on the coupling of a mechanical medium with an electrical waveguide via piezoelectric elements. The exchange of energy between the two media in correspondence with the coincidence frequency leads to substantial attenuation of waves over a fairly wide frequency range.
Vibration suppression of plates by optimally calibrated piezoelectric RL shunt damping
Johan Frederik Toftekær
PhD student, Section of Solid Mechanics, Department of Mechanical Engineering,
Danmarks Tekniske Universitet (DTU), Kongens Lyngby, Denmark
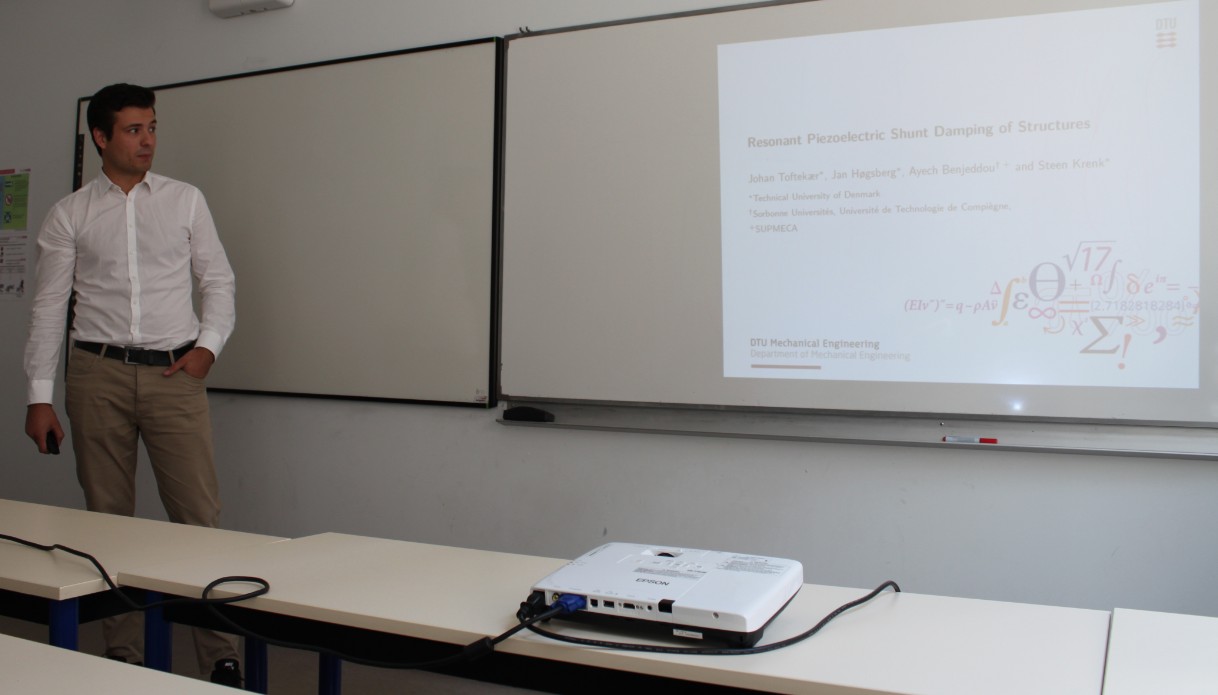
The presented work concerns vibration suppression of plates and plate-like structures, through resonant piezoelectric damping, introduced by inductive-resistive (RL) shunts, for which the performance relies on the precise calibration of the shunt frequency. An important aspect of passive shunt damping of flexible structures, like plates, thus lays in the ability to account for the energy spill-over from the non-resonant modes, which is not taken into account by common calibration methods. A newly proposed calibration procedure includes the contribution from the non-resonant modes by a quasi-dynamic modal correction, taking both flexibility and inertia effects from the residual modes into account.
In the presented work this procedure is implemented in a finite element model using Kirchhoff plate kinematics for the host structure, and a plane-stress assumption for the bonded piezoelectric patches. The established model has for the first time allowed for the comparison between the present shunt circuit calibration and results obtained from earlier proposed methods found in the literature, for benchmark examples. It is particularly found, that the calibration of the inductance is largely influenced by the contribution from the non-resonant modes, and that omission therefore leads to significant detuning of the shunt circuit, which can be seen in frequency response plots and by determination of the attained attenuations.
Controllable wave propagation with interconnected LC shunts
Edgar Flores Parra
PhD student, Electro-Mechanical Material Systems Group, Laboratory of Composite Materials and Adaptive Structures,
Institute of Design, Materials and Fabrication (IDMF), Department of Mechanical and Process Engineering (D-MAVT), ETH Zürich, Switzerland
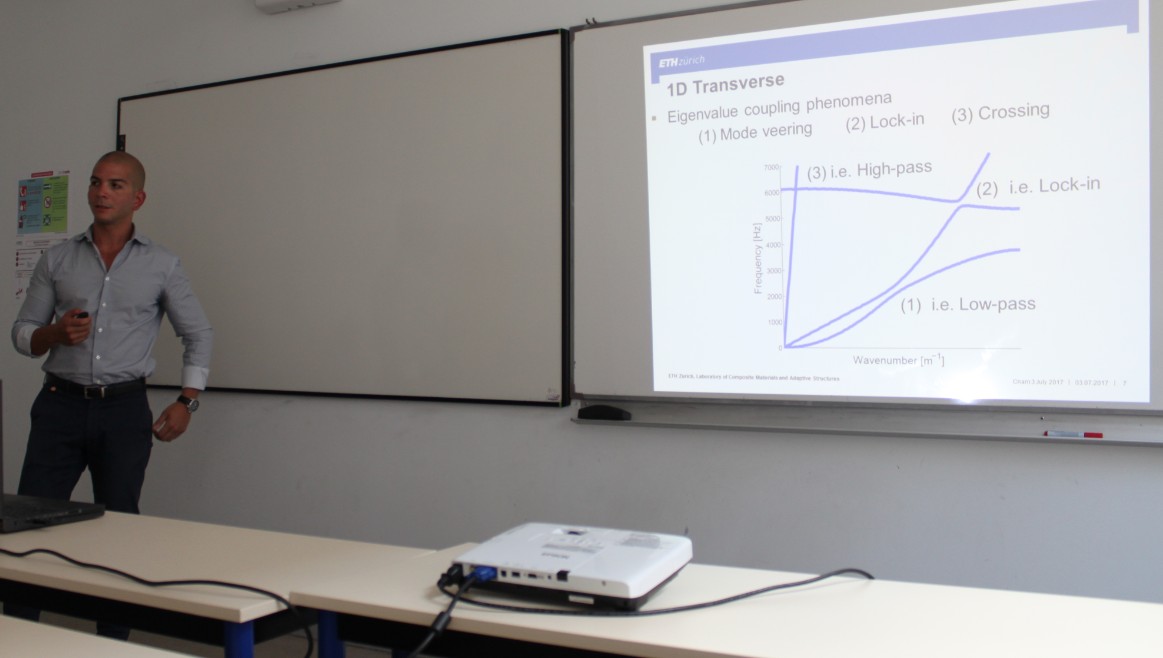
Control of propagating waves, and the ensuing vibrations can be achieved through a number of methods. One approach is the use of interconnected piezoelectric LC shunts The inductance in the LC circuit gives way to an electrical resonance as charges are exchanged with the capacitance of the piezoelectric element, while at the same time, the piezoelectric element couples the mechanical and electrical components by conversion of energy. Moreover, by interconnecting the LC shunts the design space is vastly expanded, as the latter are no longer individual components, but a secondary discrete domain through which energy can also propagate.
A myriad of interconnection schemes could be envisioned, however, this work will focus on low-pass, band-pass, high-pass filters tailored to achieve specific effects on the mechanical domain of one (1D) and two (2D) dimensional media. This work also focuses strongly on developing miniaturized programmable technologies to achieve full integration of the electrical and mechanical domains within the unit cell. As a result, truly "smart" materials with the potential of bridging the gap between theory and practical applications are developed.